Select Medium
CLASS X CHEMISTRY CHAPTER 5
Compounds of Non Metals
LESSON OVERVIEW
Compounds of non-metals play a crucial role in various industries and natural processes, encompassing a broad spectrum of chemical and physical properties. They serve as fundamental building blocks in fields ranging from agriculture and pharmaceuticals to environmental science and industrial manufacturing. This document delves into the intricate details of key non-metal compounds such as ammonia and sulphuric acid, exploring their applications, reactions, and the principles governing their behavior in diverse settings.
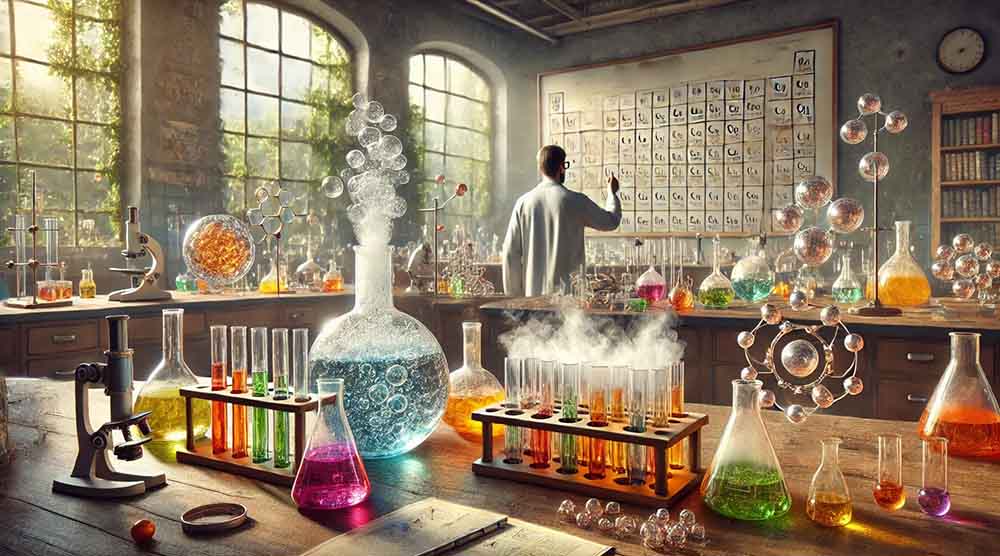
Ammonia
Description: Ammonia (NH3) is a compound of nitrogen and hydrogen. It is a colorless gas with a distinct pungent smell. Ammonia is highly soluble in water, forming ammonium hydroxide, a weak base. It is a key industrial chemical used primarily in fertilizers and is also a significant precursor for many pharmaceuticals and cleaning products.
Examples:
- Agriculture: Ammonia is used in the production of fertilizers such as ammonium nitrate and urea, which are essential for crop growth.
- Cleaning Products: Ammonia solutions are commonly used as household cleaners due to their effectiveness in removing grime and stains.
- Pharmaceuticals: Ammonia is a building block in the synthesis of various pharmaceuticals, including sulfa drugs.
Additional Information: Ammonia is produced on a large scale by the Haber-Bosch process, where nitrogen from the air is combined with hydrogen under high pressure and temperature in the presence of a catalyst. Ammonia is also used in the production of nitric acid via the Ostwald process, and as a refrigerant in industrial refrigeration systems due to its high energy efficiency and low environmental impact.
Tip for Easy Remembering: Remember ammonia as the “NHH” molecule – Nitrogen with triple Hydrogen, used in fertilizers to help plants “NHH-grow.”
Acidic and Base Properties of Gas
Description: Gases can exhibit acidic or basic properties when dissolved in water or when they react with other substances. Acidic gases, like carbon dioxide (CO2) and sulfur dioxide (SO2), form acids when dissolved in water. Basic gases, such as ammonia (NH3), form bases when dissolved.
Examples:
- Carbon Dioxide (CO2): Dissolves in water to form carbonic acid (H2CO3), which is a weak acid.
- Sulfur Dioxide (SO2): Dissolves in water to form sulfurous acid (H2SO3), which can further oxidize to form sulfuric acid (H2SO4).
- Ammonia (NH3): Dissolves in water to form ammonium hydroxide (NH4OH), a weak base.
Additional Information: The pH scale measures the acidity or basicity of a solution. Gases that form acids in water will lower the pH of the solution, while those forming bases will raise the pH. Understanding the behavior of gases in water is crucial for environmental chemistry, particularly in understanding acid rain and its effects on ecosystems.
Tip for Easy Remembering: Think of acidic gases as those that “fizz” (like CO2 in soda) and basic gases as those that “clean” (like NH3 in cleaners).
Haber Process
Description: The Haber process, or Haber-Bosch process, is an industrial method for synthesizing ammonia from nitrogen and hydrogen gases. This process is essential for the mass production of fertilizers, which support global agriculture.
Examples:
- Fertilizer Production: The ammonia produced is primarily used to manufacture fertilizers such as urea and ammonium nitrate.
- Explosives: Ammonia is also a precursor in the production of explosives like TNT and nitroglycerin.
- Refrigeration: Ammonia is used as a refrigerant gas in large-scale refrigeration systems.
Additional Information: The Haber process involves the reaction of nitrogen (N2) from the air with hydrogen (H2) derived from natural gas (methane) under high pressure and temperature (200-400°C) in the presence of an iron catalyst. The reaction is exothermic and reaches equilibrium, with the ammonia continuously removed to drive the reaction forward.
Tip for Easy Remembering: Associate the Haber process with “NH3 Harvest” – Nitrogen and Hydrogen combine to harvest ammonia for fertilizers.
Reversible Reactions
Description: Reversible reactions are chemical reactions that can proceed in both forward and backward directions. At equilibrium, the rate of the forward reaction equals the rate of the backward reaction, leading to a constant concentration of reactants and products.
Examples:
- Ammonia Synthesis (Haber Process): N2 + 3H2 ⇌ 2NH3
- Water Formation and Decomposition: 2H2 + O2 ⇌ 2H2O
- Esterification: CH3COOH + C2H5OH ⇌ CH3COOC2H5 + H2O
Additional Information: Reversible reactions are fundamental in chemical equilibrium and many biological processes, such as cellular respiration and photosynthesis. Understanding the dynamics of reversible reactions is crucial in optimizing industrial chemical processes and in developing strategies for controlling reaction outcomes.
Tip for Easy Remembering: Think of reversible reactions as a “chemical seesaw” – they can go both ways and balance at equilibrium.
Forward Reactions
Description: Forward reactions refer to the process where reactants convert into products in a chemical reaction. It is one part of a reversible reaction, typically represented by the forward arrow in a chemical equation.
Examples:
- Combustion: CH4 + 2O2 → CO2 + 2H2O
- Photosynthesis: 6CO2 + 6H2O → C6H12O6 + 6O2
- Ammonia Synthesis (Haber Process): N2 + 3H2 → 2NH3
Additional Information: The rate of the forward reaction depends on factors such as temperature, pressure, concentration of reactants, and the presence of a catalyst. In industrial processes, conditions are often optimized to favor the forward reaction to maximize product yield.
Tip for Easy Remembering: Remember forward reactions as “React to Produce” – reactants move forward to create products.
Backward Reactions
Description: Backward reactions, also known as reverse reactions, are the process where products convert back into reactants in a chemical reaction. This process is the reverse part of a reversible reaction.
Examples:
- Decomposition of Ammonia: 2NH3 → N2 + 3H2
- Decomposition of Water: 2H2O → 2H2 + O2
- Hydrolysis of Esters: CH3COOC2H5 + H2O → CH3COOH + C2H5OH
Additional Information: The backward reaction occurs simultaneously with the forward reaction in a reversible reaction system. The extent to which a reaction proceeds in the backward direction depends on the equilibrium position and the conditions of the system. In industrial applications, understanding and controlling the backward reaction is crucial for optimizing product yields.
Tip for Easy Remembering: Think of backward reactions as “Products to React” – products move backward to reform reactants.
Chemical Equilibrium
Description: Chemical equilibrium is the state in a reversible reaction where the rates of the forward and backward reactions are equal, resulting in constant concentrations of reactants and products. It represents a dynamic balance where the reactions continue to occur but without net change in concentrations.
Examples:
- Ammonia Synthesis (Haber Process): N2 + 3H2 ⇌ 2NH3
- Acetic Acid Esterification: CH3COOH + C2H5OH ⇌ CH3COOC2H5 + H2O
- Carbon Dioxide in Water: CO2 + H2O ⇌ H2CO3 ⇌ H+ + HCO3-
Additional Information: Chemical equilibrium is described by the equilibrium constant (K), which quantifies the ratio of product concentrations to reactant concentrations at equilibrium. Factors affecting equilibrium include changes in concentration, pressure, temperature, and the presence of catalysts. Le Chatelier’s principle predicts how a system at equilibrium responds to disturbances, guiding the optimization of industrial processes and understanding of biological systems.
Tip for Easy Remembering: Remember chemical equilibrium as “Balanced Reactions” – reactants and products are in a dynamic balance.
Le Chatelier’s Principle
Description: Le Chatelier’s principle states that if a system at equilibrium is disturbed by changing conditions such as concentration, temperature, or pressure, the system will shift its equilibrium position to counteract the disturbance and restore equilibrium.
Examples:
- Increase in Reactant Concentration: Adding more N2 to the Haber process shifts the equilibrium towards producing more NH3.
- Increase in Temperature: For an exothermic reaction like the Haber process, increasing temperature shifts equilibrium towards the reactants.
- Increase in Pressure: For reactions involving gases, increasing pressure shifts equilibrium towards the side with fewer gas molecules.
Additional Information: Le Chatelier’s principle is fundamental in predicting how changes in conditions affect chemical reactions and equilibrium. It helps in designing chemical processes to maximize product yields, such as in ammonia synthesis or esterification. Understanding this principle also aids in the development of strategies for controlling reactions in various industrial and laboratory settings.
Tip for Easy Remembering: Think of Le Chatelier’s principle as “Shift to Balance” – the system shifts to counteract changes and maintain equilibrium.
Influence of Concentration on Equilibrium
Description: The concentration of reactants and products can significantly influence the position of equilibrium in a reversible reaction. According to Le Chatelier’s principle, changing the concentration of reactants or products will shift the equilibrium to counteract the change.
Examples:
- Addition of Reactants: Increasing the concentration of reactants shifts the equilibrium towards the products.
- Removal of Products: Decreasing the concentration of products shifts the equilibrium towards the products.
- Ammonia Synthesis: Adding more nitrogen or hydrogen shifts the equilibrium towards producing more ammonia.
Additional Information: Changes in concentration are a common method for manipulating the position of equilibrium in industrial chemical processes. For example, in the Haber process, removing ammonia from the reaction mixture as it forms shifts the equilibrium towards the products, increasing ammonia production. Understanding how concentration affects equilibrium is essential for optimizing reaction conditions and maximizing yields in chemical manufacturing.
Tip for Easy Remembering: Remember concentration’s influence on equilibrium as “Add to Shift” – adding reactants or removing products shifts equilibrium towards the other side.
Pressure and Chemical Equilibrium
Description: Pressure affects the equilibrium position of reactions involving gases. According to Le Chatelier’s principle, changing the pressure of a system at equilibrium will shift the equilibrium position to counteract the change.
Examples:
- Haber Process: Increasing pressure shifts the equilibrium towards producing more ammonia, as fewer gas molecules are on the product side.
- Formation of Nitrogen Dioxide: 2NO + O2 ⇌ 2NO2; increasing pressure shifts equilibrium towards NO2.
- Decomposition of Ammonium Dichromate: (NH4)2Cr2O7 ⇌ N2 + 4H2O + Cr2O3; increasing pressure shifts equilibrium towards the reactants.
Additional Information: In industrial processes, controlling pressure is a key strategy for optimizing chemical reactions. For instance, the Haber process is conducted at high pressures to maximize ammonia yield. Understanding how pressure affects equilibrium is crucial for designing efficient chemical processes, particularly those involving gas-phase reactions.
Tip for Easy Remembering: Think of pressure’s influence on equilibrium as “Push to Fewer” – increasing pressure shifts equilibrium towards the side with fewer gas molecules.
Temperature and Equilibrium
Description: Temperature changes can significantly impact the equilibrium position of a reaction. According to Le Chatelier’s principle, increasing or decreasing temperature will shift the equilibrium to counteract the change in temperature.
Examples:
- Exothermic Reactions: Increasing temperature shifts equilibrium towards the reactants. Example: N2 + 3H2 ⇌ 2NH3 (Haber process).
- Endothermic Reactions: Increasing temperature shifts equilibrium towards the products. Example: N2O4 ⇌ 2NO2.
- Decomposition of Calcium Carbonate: CaCO3 ⇌ CaO + CO2; increasing temperature shifts equilibrium towards CaO and CO2.
Additional Information: Temperature control is crucial in many industrial processes to optimize reaction yields and efficiency. For example, the production of ammonia in the Haber process is carried out at an optimal temperature that balances the rate of reaction and yield. Understanding how temperature affects equilibrium helps in developing strategies for process optimization and energy management.
Tip for Easy Remembering: Remember temperature’s influence on equilibrium as “Heat Shifts” – increasing temperature shifts equilibrium towards endothermic reactions and vice versa.
Catalyst and Chemical Equilibrium
Description: A catalyst is a substance that increases the rate of a chemical reaction without being consumed in the process. Catalysts work by providing an alternative pathway for the reaction with a lower activation energy. They do not affect the position of equilibrium but help the system reach equilibrium faster.
Examples:
- Haber Process: Iron catalyst speeds up the synthesis of ammonia.
- Ostwald Process: Platinum catalyst speeds up the oxidation of ammonia to produce nitric acid.
- Catalytic Converters: Platinum and rhodium catalysts reduce vehicle emissions by converting harmful gases into less harmful substances.
Additional Information: Catalysts are essential in many industrial processes to increase reaction rates and improve efficiency. They play a critical role in environmental applications, such as reducing emissions from vehicles and industrial processes. Understanding how catalysts work and their impact on reaction rates is fundamental in chemical engineering and environmental science.
Tip for Easy Remembering: Think of catalysts as “Speed Boosters” – they speed up reactions without changing equilibrium positions.
Sulphuric Acid
Description: Sulphuric acid (H2SO4) is a highly corrosive strong acid. It is one of the most important industrial chemicals and is used in various applications, including fertilizer production, mineral processing, petroleum refining, and chemical synthesis.
Examples:
- Fertilizer Production: Used to manufacture superphosphate and ammonium sulphate.
- Battery Acid: Used in lead-acid batteries for cars.
- Industrial Cleaning: Used to clean metal surfaces before electroplating.
Additional Information: Sulphuric acid is produced industrially by the Contact process, which involves the oxidation of sulfur dioxide to sulfur trioxide, followed by absorption in water. Its properties, such as being a strong dehydrating agent and its ability to act as an oxidizing agent, make it versatile for various industrial applications.
Tip for Easy Remembering: Remember sulphuric acid as “H2SO4 Strong” – a strong acid with diverse industrial uses.
Industrial Preparation of Sulphuric Acid
Description: The industrial preparation of sulphuric acid is primarily carried out using the Contact process. This process involves several steps, including the combustion of sulfur, the formation of sulfur dioxide, its oxidation to sulfur trioxide, and the absorption of sulfur trioxide in water to form sulphuric acid.
Examples:
- Sulfur Combustion: S + O2 → SO2
- Oxidation of Sulfur Dioxide: 2SO2 + O2 ⇌ 2SO3 (catalyzed by vanadium(V) oxide)
- Formation of Sulphuric Acid: SO3 + H2O → H2SO4
Additional Information: The Contact process is highly efficient and produces concentrated sulphuric acid. The use of a catalyst (vanadium(V) oxide) in the oxidation step is crucial for achieving high conversion rates. The process also includes steps to manage heat generated during the reactions and to ensure the removal of impurities.
Tip for Easy Remembering: Think of the Contact process as “Sulfur to SO3 to H2SO4” – sulfur transforms to sulphuric acid through oxidation and hydration.
Physical and Chemical Properties of Sulphuric Acid
Dehydrating Nature: Sulphuric acid is a powerful dehydrating agent, meaning it can remove water from other substances.
Examples:
- Dehydration of Sugar: C12H22O11 + H2SO4 → 12C + 11H2O
- Dehydration of Ethanol: C2H5OH → C2H4 + H2O (catalyzed by H2SO4)
Drying Nature: Sulphuric acid is used as a drying agent to remove water from gases and liquids.
Examples:
- Drying of Gases: Used in drying towers to remove moisture from gases like hydrogen chloride.
- Desiccants: Used in desiccators to keep samples dry.
Reaction with Salts: Sulphuric acid reacts with salts to produce corresponding sulphates.
Examples:
- Reaction with Sodium Chloride: NaCl + H2SO4 → NaHSO4 + HCl
- Reaction with Calcium Carbonate: CaCO3 + H2SO4 → CaSO4 + CO2 + H2O
Oxidizing Nature: Sulphuric acid can act as an oxidizing agent, particularly at high concentrations.
Examples:
- Oxidation of Copper: Cu + 2H2SO4 → CuSO4 + SO2 + 2H2O
- Oxidation of Sulfur: S + 2H2SO4 → 3SO2 + 2H2O
Additional Information: The diverse properties of sulphuric acid make it invaluable in many industrial processes, from chemical synthesis to waste treatment. Its ability to act as a dehydrating and oxidizing agent enhances its versatility.
Tip for Easy Remembering: Think of sulphuric acid as “H2SO4 Versatile” – with properties that dehydrate, dry, and oxidize.
Identification of Sulphate Ions
Description: Identifying sulphate ions (SO4^2-) involves specific tests that confirm their presence in a solution. The most common method is the precipitation reaction with barium chloride (BaCl2).
Examples:
- Precipitation Test: Adding BaCl2 to a solution suspected of containing sulphate ions results in the formation of a white precipitate of barium sulphate (BaSO4).
- Confirmatory Test: The precipitate does not dissolve in dilute hydrochloric acid (HCl), confirming the presence of sulphate ions.
Additional Information: This test is specific and reliable for detecting sulphate ions in various samples, including water and soil. The formation of barium sulphate is a clear indication of sulphates due to its low solubility in water and dilute acids.
Tip for Easy Remembering: Remember sulphate ion identification as “BaSO4 White” – the white precipitate confirms sulphate ions.
Chemical Reactions Connected to Sulphuric Acid and Sulphate Ions
Description: Sulphuric acid and sulphate ions participate in numerous chemical reactions, including acid-base reactions, redox reactions, and precipitation reactions.
Examples:
- Reaction with Metals: Zn + H2SO4 → ZnSO4 + H2 (acid-base reaction)
2NaOH → Na2SO4 + 2H2O (neutralization) 3. Precipitation with Barium: BaCl2 + Na2SO4 → BaSO4 (precipitation)
Additional Information: These reactions are crucial in industrial processes such as metal processing, waste treatment, and analytical chemistry. Understanding the behavior of sulphuric acid and sulphate ions in these reactions helps in developing effective strategies for material processing, environmental management, and chemical analysis.
Tip for Easy Remembering: Think of reactions with sulphuric acid as “H2SO4 Reactions” – versatile in acid-base, redox, and precipitation processes.
Key Points to Remember
Ammonia (NH3)
- Used in fertilizer and cleaning product manufacture.
- Example: Synthesized industrially via the Haber process.
Acidic and Basic Properties of Gases
- Acidic gases like CO2 form acids when dissolved in water.
- Basic gases like NH3 form bases.
- Example: CO2 forms carbonic acid in water.
Haber Process
- Industrial method to synthesize ammonia from nitrogen and hydrogen.
- Example: Essential for producing ammonia-based fertilizers.
Reversible Reactions
- Reactions that can proceed in both forward and backward directions.
- Example: Water formation, 2H2+O2⇌2H2O2H_2 + O_2 \rightleftharpoons 2H_2O2H2+O2⇌2H2O.
Chemical Equilibrium
- State where the rates of the forward and reverse reactions are equal.
- Example: Ammonia synthesis in the Haber process.
Le Chatelier’s Principle
- System adjusts to minimize change affecting equilibrium.
- Example: Adding more reactant shifts equilibrium towards more products.
Influence of Concentration on Equilibrium
- Changing reactant/product concentration shifts equilibrium.
- Example: Increasing reactants in the Haber process increases ammonia production.
Pressure and Chemical Equilibrium
- Changes in pressure affect gaseous reactions’ equilibria.
- Example: Increasing pressure favors formation of fewer gas molecules (ammonia synthesis).
Temperature and Equilibrium
- Temperature affects equilibrium; endothermic vs. exothermic reactions respond differently.
- Example: Increasing temperature shifts exothermic reactions to favor reactants.
Catalysts in Chemical Reactions
- Speed up reactions without being consumed; do not shift equilibrium.
- Example: Iron catalysts in the Haber process.
Sulphuric Acid (H2SO4)
- A strong acid used widely in industry.
- Example: Manufacturing fertilizers and in lead-acid batteries.
Industrial Preparation of Sulphuric Acid
- Primarily via the Contact process.
- Example: Oxidation of sulfur dioxide to sulfur trioxide, then conversion to sulfuric acid.
Physical and Chemical Properties of Sulphuric Acid
- Strong dehydrating and oxidizing agent.
- Example: Dehydration of sugar to carbon and water.
Identification of Sulphate Ions
- Barium chloride test forms a white precipitate of barium sulphate.
- Example: Testing water samples for sulfate contamination.
Chemical Reactions Involving Sulphuric Acid and Sulphate Ions
- Reacts with various substances forming different products.
- Example: Reaction with copper oxide forms copper sulfate.
Most Predicted Questions
The practice quizzes on SSLC A+ GENIUS feature the most predicted questions for SSLC exams, complete with detailed answers and tips. These interactive quizzes are designed to enhance your memory and performance, making exam preparation more effective and enjoyable.