Select Medium
CLASS X BIOLOGY CHAPTER 6
Unravelling Genetic Mysteries
LESSON OVERVIEW
Genetics is the study of heredity and variation, originating from Gregor Mendel’s work with pea plants, which established the foundational laws of inheritance. DNA, structured as a double helix, carries genetic instructions, while genes and alleles determine traits passed from parents to offspring. Processes like hybridization, crossing over, and mutations contribute to genetic diversity, which is crucial for evolution and adaptation.
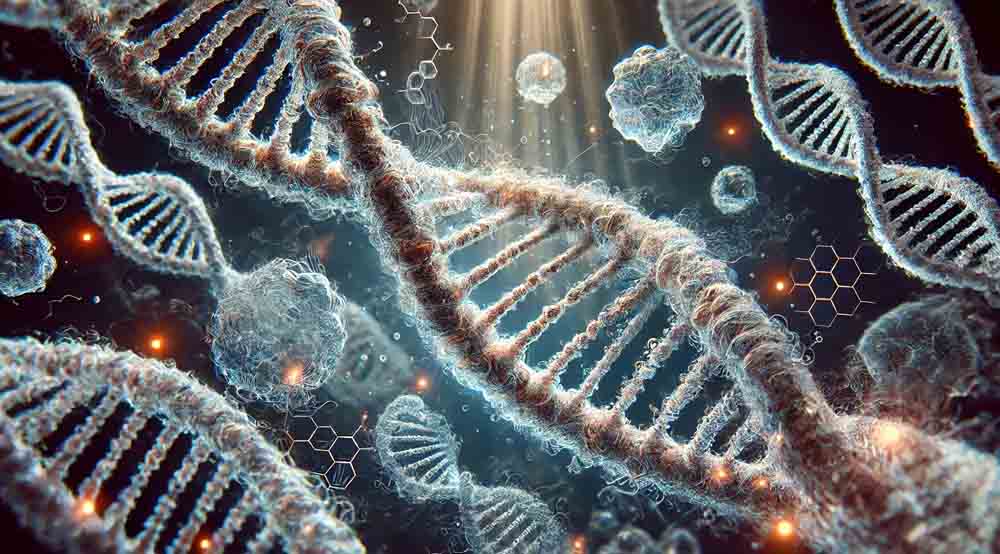
1. Emergence of Genetics
Description:
Genetics is the branch of biology that studies heredity and variation in organisms. The emergence of genetics as a scientific discipline began with Gregor Mendel, an Austrian monk who conducted groundbreaking experiments with pea plants in the mid-19th century. Mendel’s work, published in 1866, laid the foundation for the laws of inheritance, although it wasn’t recognized until the early 20th century. The rediscovery of Mendel’s work by scientists such as Hugo de Vries, Carl Correns, and Erich von Tschermak in 1900 marked the official birth of modern genetics.
Examples:
Mendel’s experiments with pea plants (Pisum sativum) led to the discovery of dominant and recessive traits, and the formulation of what we now call Mendel’s Laws: the Law of Segregation and the Law of Independent Assortment. These laws explain how traits are inherited from one generation to the next.
Additional Information:
After Mendel’s work was rediscovered, the field of genetics rapidly expanded. Thomas Hunt Morgan’s work with fruit flies (Drosophila melanogaster) in the early 1900s provided further insights into the behavior of chromosomes during inheritance. The discovery of DNA as the genetic material in the mid-20th century by scientists such as James Watson, Francis Crick, Maurice Wilkins, and Rosalind Franklin revolutionized our understanding of genetics.
Tip for Remembering:
Remember “Mendel’s Peas Paved the Path” – Mendel’s experiments with pea plants paved the way for the emergence of genetics as a science.
2. Hybridization
Description:
Hybridization refers to the process of crossing two genetically different individuals to produce offspring that inherit traits from both parents. This process can occur naturally or be artificially induced by plant or animal breeders. The resulting offspring are known as hybrids and often exhibit characteristics that are a mix of both parent species.
Examples:
A common example of hybridization in plants is the creation of hybrid corn, where different strains of corn are crossbred to produce a crop that has desirable traits such as higher yield or disease resistance. In animals, a mule is a well-known hybrid, produced by crossing a horse with a donkey.
Additional Information:
Hybridization is widely used in agriculture to improve crop varieties. Hybrids often display hybrid vigor or heterosis, where the offspring exhibit superior qualities compared to their parents. However, hybrids can also be sterile or have reduced fertility, as seen in mules.
Tip for Remembering:
Think of “Hybrid Cars and Hybrid Corn” – Just as hybrid cars combine features from different car technologies, hybridization in biology combines traits from different species or varieties.
3. Genes and Alleles
Description:
Genes are the basic units of heredity, composed of DNA, and located on chromosomes. They encode the instructions for the synthesis of proteins, which carry out various functions in an organism. Alleles are different versions of a gene that arise through mutations and can produce variations in the trait controlled by the gene.
Examples:
For instance, the gene for flower color in pea plants has two alleles – one for purple flowers and one for white flowers. An individual plant can have two identical alleles (homozygous) or two different alleles (heterozygous) for a particular gene.
Additional Information:
The concept of dominant and recessive alleles is crucial in understanding how traits are inherited. Dominant alleles mask the effect of recessive alleles in a heterozygous individual. The combination of alleles that an individual has for a particular gene is called its genotype, while the observable trait is called the phenotype.
Tip for Remembering:
Remember “Alleles Are Alternatives” – Alleles are alternative forms of a gene, each coding for a different version of the same trait.
4. Self-Pollination of First Generation Plant
Description:
Self-pollination occurs when pollen from a flower fertilizes the ovules of the same flower or another flower on the same plant. In the context of Mendel’s experiments, self-pollination of the first-generation (F1) hybrids leads to the production of the second-generation (F2) plants.
Examples:
In Mendel’s pea plant experiments, he crossed true-breeding tall plants with true-breeding short plants. The F1 generation, which was all tall, was then self-pollinated to produce the F2 generation. The resulting F2 generation exhibited both tall and short plants in a 3:1 ratio, demonstrating the segregation of alleles.
Additional Information:
Self-pollination helps in maintaining genetic purity and stability in plants but limits genetic diversity. However, it is a useful tool in controlled genetic experiments where the aim is to study the inheritance of specific traits.
Tip for Remembering:
Think “Self-Pollination Stays Same” – Self-pollination often keeps genetic traits consistent within the same plant or line, similar to how self-reflection leads to consistency in thought.
5. Hybridization Experiment and Ratio
Description:
Mendel’s hybridization experiments involved crossing different varieties of pea plants to study the inheritance of traits. His most famous experiment was the monohybrid cross, where he crossed plants differing in a single trait (e.g., flower color). The F1 generation all showed the dominant trait, but when these F1 plants were self-pollinated, the F2 generation showed a 3:1 ratio of dominant to recessive traits.
Examples:
In a monohybrid cross involving flower color, Mendel crossed a true-breeding purple-flowered plant with a true-breeding white-flowered plant. The F1 generation had all purple flowers. Self-pollination of these F1 plants resulted in an F2 generation with a 3:1 ratio of purple to white flowers.
Additional Information:
The 3:1 ratio observed in the F2 generation is a hallmark of Mendelian inheritance and reflects the segregation of alleles during gamete formation. This ratio is explained by the Law of Segregation, where each parent contributes one allele to the offspring.
Tip for Remembering:
“Three to One in F2 is Fun” – The 3:1 ratio in the F2 generation is a key result of Mendel’s experiments and can be remembered as a fun fact of genetics.
6. Hybridization of Two Characters (Dihybrid Cross)
Description:
A dihybrid cross involves hybridization between two organisms that differ in two traits. Mendel’s experiments with dihybrid crosses revealed that the inheritance of one trait does not affect the inheritance of another, leading to the formulation of the Law of Independent Assortment.
Examples:
Mendel crossed pea plants that differed in two traits, such as seed shape (round or wrinkled) and seed color (yellow or green). The F1 generation showed both dominant traits (round and yellow), but the F2 generation exhibited a 9:3:3:1 ratio of the four possible combinations of traits (round yellow, round green, wrinkled yellow, and wrinkled green).
Additional Information:
The 9:3:3:1 ratio in a dihybrid cross is a classic example of independent assortment, where alleles for different traits segregate independently during gamete formation. This principle is fundamental to understanding how multiple traits are inherited.
Tip for Remembering:
“Nine-Three-Three-One, Independent Traits Run” – The 9:3:3:1 ratio in dihybrid crosses demonstrates that traits are inherited independently of each other.
7. DNA (Deoxyribonucleic Acid)
Description:
DNA, or Deoxyribonucleic Acid, is the molecule that carries the genetic instructions for the development, functioning, growth, and reproduction of all known living organisms and many viruses. DNA is a long polymer made of repeating units called nucleotides, each consisting of a sugar, a phosphate group, and a nitrogenous base.
Examples:
DNA is found in the nucleus of eukaryotic cells and in the cytoplasm of prokaryotic cells. It encodes the information necessary to build and maintain an organism. For example, the DNA sequence in a human cell contains instructions for making proteins, which perform various functions like catalyzing metabolic reactions and providing structural support.
Additional Information:
The structure of DNA was first described by James Watson and Francis Crick in 1953 as a double helix, where two strands are wound around each other, with base pairs (adenine with thymine and cytosine with guanine) forming the rungs of the helix. DNA replication, transcription, and translation are key processes in the central dogma of molecular biology.
Tip for Remembering:
“DNA is the Blueprint of Life” – DNA holds the instructions for everything in living organisms, just like a blueprint guides the construction of a building.
8. Structure of DNA
Description:
The structure of DNA is often described as a double helix, resembling a twisted ladder. The sides of the ladder are made of alternating sugar (deoxyribose) and phosphate molecules, and the rungs are made of nitrogenous base pairs held together by hydrogen bonds. The two strands of the helix run in opposite directions (antiparallel).
Examples:
In the DNA double helix, adenine (A) pairs with thymine (T), and cytosine (C) pairs with guanine (G). This complementary base pairing is essential for the accurate replication of DNA during cell division and for the transcription of genetic information into RNA.
Additional Information:
The antiparallel nature of DNA strands is crucial for the replication process, where enzymes like DNA polymerase can only add nucleotides to the 3′ end of a growing DNA strand. The double helix structure also allows DNA to be tightly packed into chromosomes, facilitating its storage in the nucleus.
Tip for Remembering:
“Double Helix, Twisted Bliss” – The double helix is the iconic structure of DNA, with its twisted ladder-like form ensuring stability and accurate replication.
9. Nucleotide
Description:
Nucleotides are the building blocks of DNA and RNA. Each nucleotide consists of three components: a phosphate group, a five-carbon sugar (deoxyribose in DNA, ribose in RNA), and a nitrogenous base (adenine, thymine, cytosine, or guanine in DNA; uracil replaces thymine in RNA).
Examples:
In DNA, nucleotides link together to form long chains, with the phosphate group of one nucleotide bonding to the sugar of the next, creating a sugar-phosphate backbone. The sequence of nitrogenous bases along the DNA strand encodes genetic information.
Additional Information:
Nucleotides also play critical roles in cellular metabolism and signaling. For example, ATP (adenosine triphosphate) is a nucleotide that serves as the primary energy carrier in cells. NAD+ (nicotinamide adenine dinucleotide) is another nucleotide involved in redox reactions.
Tip for Remembering:
“Nucleotides: The ABCs of DNA” – Just as the alphabet is made up of letters, DNA is made up of nucleotides, which are the fundamental units of its structure.
10. Strands of DNA
Description:
DNA is composed of two long strands that run in opposite directions (antiparallel) and are held together by hydrogen bonds between paired nitrogenous bases. Each strand has a 5′ end (with a phosphate group) and a 3′ end (with a hydroxyl group), and the two strands form a double helix.
Examples:
In the double helix, one strand runs from 5′ to 3′, while the complementary strand runs from 3′ to 5′. This arrangement is crucial for processes like DNA replication, where the enzyme DNA polymerase adds nucleotides to the 3′ end of a growing strand.
Additional Information:
The antiparallel nature of DNA strands ensures that the genetic code is read in the correct direction during transcription and translation. This directional reading is essential for the accurate synthesis of proteins and the expression of genes.
Tip for Remembering:
“Antiparallel Strands, Opposite Ends” – DNA strands run in opposite directions, which is key to their function and replication.
11. RNA (Ribonucleic Acid)
Description:
RNA, or Ribonucleic Acid, is a single-stranded molecule that plays a vital role in coding, decoding, regulation, and expression of genes. RNA is similar to DNA but has ribose as its sugar and uracil instead of thymine as one of its bases.
Examples:
There are several types of RNA, each with specific functions:
mRNA (messenger RNA): Carries the genetic code from DNA to the ribosome for protein synthesis.
tRNA (transfer RNA): Brings amino acids to the ribosome during protein synthesis.
rRNA (ribosomal RNA): Combines with proteins to form ribosomes, the site of protein synthesis.
Additional Information:
RNA is involved in various cellular processes, including transcription (copying DNA into RNA), translation (using mRNA to synthesize proteins), and gene regulation. RNA can also have catalytic functions, as seen in ribozymes, which are RNA molecules that catalyze chemical reactions.
Tip for Remembering:
“RNA: The Messenger and More” – RNA acts as the messenger carrying instructions from DNA, but it also has many other roles in the cell.
12. Action of Genes
Description:
Genes exert their effects by being transcribed into RNA, which is then translated into proteins. These proteins carry out various functions within the cell, including catalyzing metabolic reactions, providing structural support, and regulating gene expression.
Examples:
The gene for hemoglobin, a protein in red blood cells, is transcribed into mRNA, which is then translated into the hemoglobin protein. Hemoglobin binds oxygen and transports it throughout the body.
Additional Information:
Gene expression is tightly regulated, with various factors controlling when and how much a gene is transcribed and translated. Mutations in genes can lead to the production of defective proteins, causing genetic disorders.
Tip for Remembering:
“Genes Act by Making Proteins” – Genes express their function through the production of proteins, which perform the essential tasks in the cell.
13. Chromosomes in Humans
Description:
Chromosomes are thread-like structures located in the nucleus of a cell that carry genetic information in the form of genes. Humans have 23 pairs of chromosomes, for a total of 46. These include 22 pairs of autosomes and one pair of sex chromosomes (XX in females, XY in males).
Examples:
Each parent contributes one chromosome to each pair, so offspring inherit half of their chromosomes from their mother and half from their father. Abnormalities in chromosome number or structure can lead to genetic disorders, such as Down syndrome, which is caused by an extra copy of chromosome 21.
Additional Information:
Chromosomes are made up of chromatin, a combination of DNA and protein. During cell division, chromosomes condense and become visible under a microscope. The centromere is the region where the two chromatids are joined, and the telomeres are the protective ends of the chromosome.
Tip for Remembering:
“Humans Have 23 Pairs” – Remember that humans have 23 pairs of chromosomes, with the 23rd pair determining the sex of the individual.
14. Genetics of Variation
Description:
Genetic variation refers to the differences in DNA sequences among individuals within a population. This variation is the result of mutations, genetic recombination during sexual reproduction, and gene flow between populations. Genetic variation is essential for evolution, as it provides the raw material for natural selection.
Examples:
In humans, genetic variation is evident in traits such as eye color, blood type, and susceptibility to diseases. The genetic differences between individuals are what make each person unique.
Additional Information:
Genetic variation can arise through several mechanisms:
Mutations: Random changes in the DNA sequence that can introduce new alleles into a population.
Recombination: The shuffling of alleles during meiosis that produces new combinations of traits.
Gene Flow: The transfer of genes between populations, which can introduce new genetic material.
Tip for Remembering:
“Variation is the Spice of Life” – Genetic variation is what makes each individual unique and is crucial for the survival and evolution of species.
15. Crossing Over in Chromosomes – A Source of Variation
Description:
Crossing over is a process that occurs during meiosis, where homologous chromosomes exchange segments of genetic material. This exchange increases genetic diversity by creating new combinations of alleles on each chromosome.
Examples:
In humans, crossing over occurs between the maternal and paternal homologous chromosomes during prophase I of meiosis. This recombination leads to offspring having a mix of traits from both parents, contributing to genetic variation within a population.
Additional Information:
Crossing over is a key source of genetic diversity in sexually reproducing organisms. It ensures that each gamete (sperm or egg) carries a unique set of genetic information, which, when combined with another gamete during fertilization, results in offspring with a unique genetic makeup.
Tip for Remembering:
“Crossing Over Creates Diversity” – The process of crossing over mixes genetic material, leading to increased genetic diversity in populations.
16. Combination of Alleles During Fertilization
Description:
Fertilization is the process by which two gametes (sperm and egg) unite to form a zygote. Each gamete carries half the number of chromosomes (haploid), and their combination restores the full number of chromosomes (diploid) in the zygote. The combination of alleles from both parents determines the genotype and phenotype of the offspring.
Examples:
If one parent contributes an allele for brown eyes (B) and the other contributes an allele for blue eyes (b), the offspring could have the genotype Bb. Since brown eyes are dominant, the offspring would have brown eyes.
Additional Information:
The combination of alleles during fertilization follows the principles of Mendelian inheritance, where dominant and recessive alleles interact to determine traits. Fertilization also contributes to genetic diversity, as it combines the genetic material from two different individuals.
Tip for Remembering:
“Fertilization is a Genetic Mix” – Fertilization combines alleles from both parents, creating a new genetic mix in the offspring.
17. Mutation
Description:
A mutation is a change in the DNA sequence that can occur spontaneously or due to environmental factors such as radiation or chemicals. Mutations can affect a single nucleotide (point mutation), involve large segments of a chromosome, or even result in the addition or loss of entire chromosomes.
Examples:
A point mutation in the hemoglobin gene can lead to sickle cell anemia, a condition where red blood cells assume an abnormal, sickle shape. This mutation affects the protein’s ability to carry oxygen efficiently.
Additional Information:
Mutations can be classified as beneficial, neutral, or harmful depending on their effect on the organism. While many mutations are neutral or harmful, some can confer advantages, such as increased resistance to diseases. Mutations are a key driver of evolution, as they introduce new genetic variation into populations.
Tip for Remembering:
“Mutation Means Change” – Mutations are changes in the DNA that can have various effects, from harmless to harmful.
18. Difference in Skin Color
Description:
Skin color is a polygenic trait, meaning it is controlled by multiple genes. These genes influence the production of melanin, the pigment responsible for skin color. The amount and type of melanin produced by melanocytes (skin cells) determine the wide range of skin colors observed in humans.
Examples:
People with darker skin have more melanin and a higher proportion of eumelanin, which provides protection against UV radiation. Those with lighter skin have less melanin and a higher proportion of pheomelanin, which provides less UV protection.
Additional Information:
Skin color is also influenced by environmental factors, such as sun exposure, which can increase melanin production and lead to tanning. The evolution of skin color is believed to be an adaptation to varying levels of UV radiation in different parts of the world, with darker skin providing protection in areas with intense sunlight and lighter skin allowing for better vitamin D synthesis in areas with lower sunlight.
Tip for Remembering:
“Melanin Makes Skin Color” – The amount and type of melanin produced by the body determine skin color.
Key Points to Remember
Emergence of Genetics:
- Originated with Gregor Mendel’s pea plant experiments.
- Mendel’s Laws: Law of Segregation and Law of Independent Assortment.
- Rediscovery in 1900 led to modern genetics.
Hybridization:
- Cross-breeding of different species/varieties to produce hybrids.
- Common in agriculture for desirable traits (e.g., hybrid corn).
Genes and Alleles:
- Genes are DNA segments coding for traits.
- Alleles are different versions of a gene.
Self-Pollination of F1 Plants:
- Leads to F2 generation with a 3:1 ratio of traits.
- Used in controlled genetic studies.
Hybridization Experiment and Ratio:
- Monohybrid cross reveals 3:1 dominant to recessive ratio in F2.
- Demonstrates Mendelian inheritance.
Hybridization of Two Characters:
- Dihybrid cross reveals a 9:3:3:1 ratio in F2.
- Shows independent assortment of traits.
DNA (Deoxyribonucleic Acid):
- Carries genetic instructions in a double helix structure.
- Composed of nucleotides: sugar, phosphate, and nitrogenous base.
Structure of DNA:
- Double helix with antiparallel strands.
- Base pairs: A-T, C-G, held by hydrogen bonds.
Nucleotide:
- Building block of DNA/RNA: phosphate, sugar, base.
- DNA has deoxyribose; RNA has ribose.
Strands of DNA:
- Two antiparallel strands form the double helix.
- Essential for replication and gene expression.
RNA (Ribonucleic Acid):
- Single-stranded, replaces thymine with uracil.
- Types: mRNA (carries code), tRNA (transfers amino acids), rRNA (forms ribosomes).
Action of Genes:
- Genes are transcribed into RNA, translated into proteins.
- Proteins perform cellular functions.
Chromosomes in Humans:
- 23 pairs, 46 total (22 autosomes, 1 pair of sex chromosomes).
- Carries genetic information.
Genetics of Variation:
- Arises from mutations, recombination, and gene flow.
- Essential for evolution and diversity.
Crossing Over in Chromosomes:
- Occurs during meiosis, increases genetic diversity.
- Homologous chromosomes exchange segments.
Combination of Alleles During Fertilization:
- Alleles from each parent combine to form the offspring’s genotype.
- Follows Mendelian inheritance patterns.
Mutation:
- Changes in DNA sequence; can be beneficial, neutral, or harmful.
- Drives evolution and genetic diversity.
Difference in Skin Color:
- Controlled by multiple genes (polygenic).
- Melanin production varies, influenced by environment.
Most Predicted Questions
The practice quizzes on SSLC A+ GENIUS feature the most predicted questions for SSLC exams, complete with detailed answers and tips. These interactive quizzes are designed to enhance your memory and performance, making exam preparation more effective and enjoyable.